Prediction of the potential global distribution of the Asian longhorned beetle Anoplophora glabripennis (Coleoptera: Cerambycidae) under climate change
Abstract
- The Asian longhorned beetle (ALB) Anoplophora glabripennis (Motschulsky) (Coleoptera: Cerambycidae) is an important wood-boring pest that has caused substantial damage to broadleaf trees in Asia, North America, and Europe.
- We used the modelling software CLIMEX to project the potential global distribution of ALB based on both historical (1987–2016) and future (2021–2050) climate conditions. ALB has possible hosts in 37 genera, and their known distributions were incorporated into the model to assess their effect on pest distribution.
- Suitable regions for ALB are predicted to be widely distributed under both historical and future climate conditions, and across all continents except Antarctica. With climate change, climate suitability would increase in the regions north of 30°N and decline in most regions south of 30°N.
- The area of most climate-suitable regions would be covered by potential hosts, and optimum hosts would dominate. The possibility of ALB outbreaks in the Northern Hemisphere is much higher than in the Southern Hemisphere, owing to the richer abundance of hosts.
- These results provide theoretical guidance for developing effective ALB monitoring and mitigation measures.
Introduction
The Asian longhorned beetle (ALB) Anoplophora glabripennis (Motschulsky) (Coleoptera: Cerambycidae) is native to China and Korea. Since the first European record of ALB was reported in Austria in 2001 (Hérard et al., 2006), local outbreaks have been continuously reported in many European countries, such as France (2003), Germany (2004), Italy (2007), Belgium (2008), the Netherlands (2010), Switzerland (2011), the United Kingdom (2012), Finland (2015), and Montenegro (2015) (Javal et al., 2019). In North America, ALB was first discovered in the United States in 1996 (Haack et al., 1996), and Canada in 2003 (Turgeon et al., 2015).
It takes ALB 1–2years to complete one generation. ALB may overwinter as egg, larvae, or pupae (Herard et al., 2009). Depending on the climate, larvae pupate from April to May, and adults emerge from mid-May to October, peaking in July. Adults mainly feed on leaf petioles and debark small branches, also feeding on the cambium. During summer or early fall, females chew an oviposition pit in the bark, and then eggs are laid beneath the bark into the phloem (Meng et al., 2015). The eggs usually hatch when the temperature exceeds 25°C (Keena, 2006), but they do not hatch if they are laid during or after September, instead these eggs overwinter and hatch when it warms up again the next year (Haack et al., 1996; Luan, 2016). As a polyphagous pest, ALB feeds on many broad-leaved tree species. The major hosts of ALB belong to the genera Populus, Salix, and Acer; minor hosts include species from Alnus, Malus, Morus, Platanus, Ulmus, and Prunus (Van der Gaag & Loomans, 2014). ALB has caused significant ecological and economic losses in more than 26 countries and is regarded globally as a quarantine pest. It is regulated by the European Union and included on the European and Mediterranean Plant Protection Organization (EPPO) A1 list (Yamaoka, 1988; Ciampitti & Cavagna, 2014). Although quarantine zones and eradication programmes have been established in most affected countries, advanced measures are urged to prevent further damage and ALB population spread (Faccoli & Gatto, 2016). Accurate projections of the potential distribution of the pest are thus needed to guide the establishment of effective preventive measures such as quarantine supervision and the management of imported solid wood packing materials.
Based on the Intergovernmental Panel on Climate Change (IPPC) Fifth Assessment Report (AR5), globally averaged combined land and ocean surface temperatures warmed by 0.85°C (0.65–1.06°C) from 1880 to 2012, and the global mean surface temperature by the end of the 21st century (2081–2100) will increase by 1.1–4.8°C (IPCC and Core Writing, 2014). Insects are poikilotherms and are highly likely to be affected by global climate change. These effects include changes of insect population densities, expanding geographical ranges, and intensified outbreak behaviours (Logan et al., 2003; Chen & Ma, 2010), and could cause unexpected ecological and economic losses by increasing the risk of invasion to new regions. In recent years, ALB's range has expanded to higher latitude and colder regions, such as Tibet and Xinjiang province in China (Huang, 2004; Yue et al., 2011).
Species distribution models (SDMs) have become increasingly important tools for research on ecology, biogeography, evolution, and climate change (Guisan & Thuiller, 2005). SDMs generally relate species distribution data with environmental factors and predict the potential distribution of a given species across a landscape (Elith & Leathwick, 2009; Sobek-Swant et al., 2012). Theoretical and technological advances have led to the development of SDMs and related software, such as CLIMEX, GARP, and Maxent (Guisan & Thuiller, 2005; Yang et al., 2013; Ge et al., 2014).
CLIMEX in particular is often used to project potential pest distributions under climate change. CLIMEX mainly describes how a species responds to climatic variables at appropriate temporal scales (daily or weekly) given a set of parameters. Compared with other models that attempt to characterize the environment occupied by a species, CLIMEX simulates the determinants of seasonal phenology, geographical distributions, and, to some extent, relative abundances (Kriticos et al., 2015). However, the model has been criticized for not considering the effects of biotic interaction: one such example is host species, which are a significant factor altering the distribution of pests (Berzitis et al., 2014). Some researchers have compensated by combining extra variables with the CLIMEX model outputs (Berzitis et al., 2014; Zou et al., 2020). For polyphagous pests like ALB, it is necessary to obtain the distribution information of all host species to comprehensively address their influence.
Previous studies have attempted to project the potential distribution of ALB using different models. For instance, Peterson and Scachetti-Pereira (2004) used the GARP ecological niche model to explore the potential distribution of ALB in North America. CLIMEX was used by MacLeod et al. (2002) to investigate suitable regions for ALB in Europe. However, the potential distribution of ALB has not been predicted at a global scale, and little is known about the influence of climate change and host plants on suitable regions for ALB.
To determine the future global potential distribution of ALB, we used CLIMEX to predict three factors under historical (1987–2016) and future (2021–2050) climate conditions: (i) the global distribution of regions climatically favourable for ALB development; (ii) the changes in the distribution and climatic suitability for ALB; (iii) how the distribution of ALB host plants affects ALB distribution. These projections can provide theoretical support for developing effective ALB population spread and reduction measures.
Materials and methods
Research model and software
CLIMEX model
We used the dynamic simulation model CLIMEX 4.0.0 (Hearne Scientific Software, Australia) to model ALB distribution. CLIMEX has been widely used in predicting the potential distribution of plants (Pattison & Mack, 2008; Shabani et al., 2012), animals (Ge et al., 2014; Zou et al., 2020), and diseases (Brasier & Scott, 1994; Yonow et al., 2013).


ArcGIS
The Spatial Analyst Module of ArcMap 10.2 (ESRI, US Environment Systems Research Institute) was used to process CLIMEX outputs. The function ‘Inverse Distance-Weighted (IDW) interpolation’ was used to convert the EI tables into raster files, and the ‘SetNull’ function in ‘raster calculator’ was used to reclassify the layers of each EI category under historical and future climate conditions. ArcGIS was also used to calculate the proportion of each continent assigned to each EI category.
Data collection
Climate data
Historical climate data were obtained for the period 1987–2016. High-resolution (0.5° × 0.5°) climate data were extracted from the gridded Climatic Research Unit (CRU, University of East Anglia) Time-series data version 4.01. Five types of meteorological data were extracted: monthly average maximum temperature, monthly average minimum temperature, average monthly rainfall, average relative humidity at 09.00 h, and average relative humidity at 15.00 h.
The period 2021–2050 was chosen for future climate simulations, to facilitate early detection and the implementation of relevant eradication measures. To obtain these future climate estimates, the multi-model ensemble mean climate dataset from 29 Global Climate Models (see Supporting information, Table S1 for model list) during the target future years (2021–2050) and the baseline period (1987–2016) was outputted to obtain climate differences under Representative Concentration Pathway (RCP) 4.5 (Zou et al., 2020). This was done for each of the above-mentioned five types of meteorological data. Then, the differences were added into the historical climate data mentioned in the last paragraph, to obtain the calibrated future climate data. The RCP4.5 scenario assumes the anthropogenic forcing will essentially level off at 4.5 W/m2 around the mid-21st century and represents the intermediate range of the four scenarios (RCP2.6, RCP4.5, RCP6.0, and RCP8.5) (Eichler et al., 2013). It was selected as its projective global mean surface temperature increase of 1.1–2.6°C was consistent with the reasonable temperature rise (1.5°C) at the end of the century (Stocker et al., 2014).
ALB distribution data
The known global distribution of ALB was mainly obtained from published literature (Yan, 1985; Haack et al., 1996; Benker & Bögel, 2006; Scheel, 2009; Meng et al., 2015; Javal et al., 2019), the Centre for Agriculture and Bioscience International (CABI) database (CABI Distribution maps of Anoplophora glabripennis), EPPO (EPPO Global DataBase Anoplophora glabripennis), and Global Biodiversity Information Facility (GBIF) database (GBIF Distribution Maps of Anoplophora glabripennis). We conducted a simple data cleaning process on the occurrence data from the GBIF website, removing the coordinates of old years (pre-1900), duplicates, or obvious errors. All occurrence records were obtained even though the pest had been eradicated in some areas. As shown in Fig. 1, the known distribution of ALB covered three continents: Asia, Europe, and North America. Detailed distribution information is listed in Supporting information, Table S2.
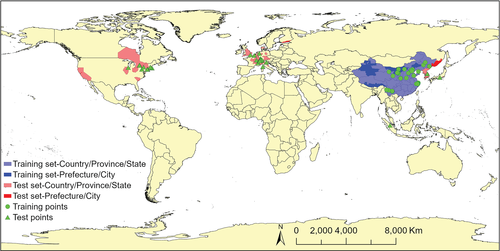
ALB host data
To capture the effect of host distribution, we conducted a thorough literature review and found 37 genera or species that acted as potential ALB hosts (see Supporting information, Table S3 for detailed classification and information sources). These were divided into three categories: (i) optimum; (ii) sub-optimum; and (iii) occasional to rare. Optimum hosts have documented evidence of ALB preference, and are trees on which ALB can complete a whole generation (e.g. trees of the Acer, Salix, Populus, Ulmus, Betula, Aesculus, and Platanus genera). Sub-optimum hosts are trees on which ALB has been reported to complete part of its life cycle or has caused damage in some areas (e.g. trees of the Albizia, Alnus, and Carpinus genera, among others). Occasional to rare hosts are tree species with little reported damage by ALB (e.g. trees in the Celtis, Hibiscus, and Rosa genera).
The distribution records of all hosts were then downloaded from the GBIF website and the data cleaned in a method similar to that of the ALB occurrence data. Hosts belonging to the same category had their distributions mapped and merged in a single layer in ArcGIS. Host occurrences (point data) were converted into a binary raster – indicating presence/absence of at least one host occurrence point per cell – that was the same resolution as our climate raster. Finally, the overlapping distribution of optimum hosts, sub-optimum hosts, and occasional to rare hosts is shown in Fig. 5(A), and the complete distribution of each was separately shown in Fig. 5(B–D).
Research methods
Parameter fitting
To obtain reasonable occurrence parameters for ALB, pest occurrence data for China were selected as the training dataset to develop the model, and data for other regions were used to validate predictive performance. The parameters were adjusted to fit the known ALB native distribution in China by referring to both published biological data and related templates within CLIMEX. The parameters were adjusted until they satisfied the following four requirements: (i) the predicted distribution covered all occurrence records of the test dataset; (ii) the climates of the predicted distribution were suitable for ALB survival; (iii) the presence of unfavourable regions (e.g. too cold, too dry) could be explained by certain ALB biological characteristics; and (iv) the predicted number of annual ALB generations was consistent with or slightly greater than the actual recorded number of generations in a given area (applicable when parameter “Minimum degree-days above DV0 necessary to complete a generation” PDD ≠ 0). The final parameters are listed in Table 1.
Parameters | Descriptions | Values for ALB |
---|---|---|
Moisture | ||
SM0 | Lower soil moisture threshold | 0.001 |
SM1 | Lower optimum soil moisture | 0.2 |
SM2 | Upper optimum soil moisture | 1.5 |
SM3 | Upper soil moisture threshold | 2.5 |
Temperature | ||
DV0 | Lower threshold (°C) | 8 |
DV1 | Lower optimum temperature (°C) | 16 |
DV2 | Upper optimum temperature (°C) | 28 |
DV3 | Upper threshold (°C) | 32 |
Cold stress | ||
TTCS | Cold stress temperature threshold (°C) | −17 |
THCS | Cold stress temperature rate (week-1) | −0.0001 |
Heat stress | ||
TTHS | Heat stress temperature threshold (°C) | 35 |
THHS | Heat stress temperature rate (week-1) | 0.1 |
Dry stress | ||
SMDS | Soil moisture dry stress threshold | 0.001 |
HDS | Stress accumulation rate (week-1) | −0.001 |
Wet stress | ||
SMWS | Soil moisture wet stress threshold | 2.5 |
HWS | Stress accumulation rate (week-1) | 0.05 |
Threshold heat sum | ||
PDD | Length of growing season (degree days) | 0 |
Temperature index
Keena (2006) and Straw et al. (2015) reported that lower threshold temperatures for ALB adult and pupal development are 5°C and 10°C, respectively. Wang et al. (2010) measured that eggs can hatch at temperatures above 7.6°C, and the lower threshold temperature for larval development is 7.77°C (Zhao et al., 1991). Therefore, the lower temperature threshold for ALB development (DV0) was adjusted in the range of 5–10°C and finally set to 8°C to fit the occurrence records in northern China.
The optimum temperature range for adult development is 16–28°C (Zhou et al., 1984), 23–24°C for adult reproduction, and the maximum hatching rate of eggs occurs at 23°C (Keena, 2006). And the optimum temperature for pupal development is 15–30°C (Straw et al., 2015). To satisfy ALB's temperature requirement in all life stages, the lower optimal temperature for ALB (DV1) and the upper optimal temperature for ALB (DV2) were set to 16°C and 28°C, respectively.
The upper threshold temperature for egg hatching is 32°C, and oviposition is arrested when the temperature exceeds 35°C (Keena, 2006). Larval development is inhibited at constant temperatures above 30°C (Keena & Moore, 2010). The upper temperature threshold for ALB development (DV3) was thus set to 32°C.
Moisture index
Soil-moisture-related stress affects pests indirectly by affecting their hosts. As soil moisture data were unavailable, relative humidity of the known ALB distribution was used in combination with templates within CLIMEX to determine soil moisture settings, and some adjustments were made to match the known distribution. For ALB, the lower moisture threshold (SM0) was set to 0.001 to satisfy its distribution records in some drought regions in Gansu, Inner Mongolia, and Ningxia, China. Large outbreaks of ALB are located in the semi-arid regions in China (Zhang & Liu, 2002; Li et al., 2011), so we refer to CLIMEX's semi-arid template and set the lower optimum soil moisture (SM1) to 0.2.
In laboratory experiments, a relative humidity of 40–60% and a temperature of <26°C are favourable for the artificial breeding of ALB (Li et al., 2017a; Li et al., 2017b), which is similar to the environments under temperate climate conditions. Also, ALB is most widely distributed in temperate climate zones in China, the upper optimum soil moisture (SM2) and the upper soil moisture threshold (SM3) were set to CLIMEX's temperate templates of 1.5 and 2.5, respectively.
Cold stress
As a freeze-tolerant species, the supercooling point (SCP) of ALB is lower than that of many other insects, and ranges from −5.98°C to −17.48°C depending on the host plant (Feng et al., 2014). Insects often die of cold stress at ambient temperatures lower than SCP, thus SCP was regarded as an important measure of ALB's cold tolerance (Ouyang & Ge, 2014). In the CLIMEX program, the cold stress temperature threshold (TTCS) defines a temperature below which cold stress begins to accumulate. The rate at which this stress accumulates is determined by the cold stress temperature rate (THCS). Cold stress would strengthen with the decrease of temperature, which is in an inverse relationship with the cold stress. For ALB, TTCS was adjusted within the range of −5.98°C to −17.48°C, and set to −17°C to account for both ALB distribution records in north-east China and the average temperature difference of 2°C between the tree phloem and the surrounding air, while phloem can provide a relatively significant buffer against cold stress (Bolstad et al., 1997). THCS was set to −0.0001/week to fit ALB distribution records in Inner Mongolia and north-eastern China.
Heat stress
The highest temperature for egg survival is 35°C (Keena & Moore, 2003). The estimated upper threshold for adult fecundity is 34–35°C, whereas adult activity is limited at temperatures exceeding 37°C (Keena, 2006). The heat stress temperature threshold (TTHS), above which heat stress accumulates, was thus set to 35°C. The heat stress accumulation rate (THHS), the rate at which heat stress accumulates, was set to 0.1/week to exclude tropical regions of China.
Dry stress
Dry stress accumulates when the soil moisture level falls below the dry stress threshold (SMDS), at the rate specified by dry stress rate (HDS). For ALB, the SMDS was fixed at the same level as SM0 (i.e. 0.001). To account for the occurrence of ALB in some dry regions of Western China, such as Gansu in Xinjiang province, HDS was adjusted to −0.001/week.
Wet stress
The wet stress threshold was set to the same value as SM3 (i.e. 2.5). The wet stress rate, the rate at which wet stress accumulates, was set to 0.05/week, this excluded some coastal Asian countries, which have a humid climate unsuitable for ALB.
Length of growing season
The Length of growing season (PDD) describes the number of degree days required to complete an entire generation (Kriticos et al., 2015). PDD is used to calculate the potential number of generations per year, and is usually a factor limiting the development of multivoltine insect species. ALB completes a generation in 1–2 years, depending on location; it is univoltine in the southern and central regions in China and bivoltine in northern China. When the thermal accumulation in the first year is insufficient for larvae to grow to the threshold weight for pupation, an additional year is required for pupation (Keena, 2006; Keena & Moore, 2010). For species that complete less than one generation per year, the EI values in CLIMEX were restricted to 0 because the thermal accumulation cannot meet the requirements for the species. PDD was thus set to 0 in our ALB model to avoid distorting effects of PDD on EI values.
Classification of EI values
EI values are an overall measure of a given location's suitability for an insect's continued survival. EI values are generally divided into categories to describe favourability, and these categories change depending on the species. However, as information on ALB's worldwide distribution in regards to climate suitability is limited, we referred to a categorization published by Sutherst (2003) to obtain the following four categories: unfavourable location (EI = 0), marginal location (0 < EI ≤ 10), favourable location (10 < EI ≤ 20), and very favourable location (EI > 20).
Analysis of results
The Spatial Analyst Module of ArcMap 10.2.2, developed by the US Environment Systems Research Institute (ESRI) (RedLands, CA) was used to analyse our results. ALB's EI values from CLIMEX were exported as raster files with the same resolution as the climate data. ArcGIS's Inverse Distance-Weighted (IDW) function was used to process these EI values, and distribution maps of unfavourable, marginal, favourable, and very favourable locations were obtained for historical (1987–2016) and future (2021–2050) climate conditions. The area proportions of different EI favourability categories under historical and future periods were also calculated.
To assess the effects of climate change on the potential distribution of ALB, we calculated the difference between EI values of historical and future climate conditions. Curves showing the difference in EI values were generated using methods outlined by Ge et al. (2019): average EI values for all locations in a given latitude were calculated, and the difference in averages between historical and future climate conditions was calculated.
The distribution maps of ALB according to host suitability were acquired by overlapping the known distribution of hosts in three categories (optimum, sub-optimum, and occasional to rare) with ALB's projected distribution under historical and future climate conditions: these climate-suitable cells containing at least one host occurrence point were regarded as ALB potential distributions.
Results
Projected potential distribution of ALB under historical and future climate conditions
Under historical climate conditions, ALB was predicted to be widely distributed on all continents except Antarctica (Fig. 2A). The predicted distribution included all distribution records in the test set, confirming that our model matches actual occurrence records with a high degree of accuracy.
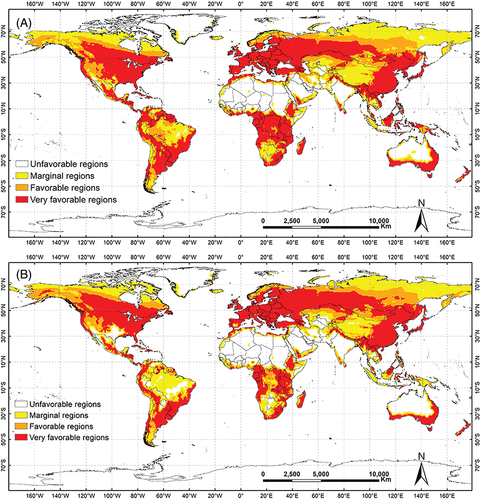
Very favourable regions accounted for 39.24% and 37.13% of the total land area (except Antarctica) under historical and future climate conditions, respectively (Fig. 3). Europe had the highest percentage of very favourable areas at 77.43% for historical and 83.4% for future climate conditions. South America followed with 49.60% for historical and 33.98% for future, and other continents clustered around 35% for both climate conditions. Within continents, very favourable regions were mainly located in Europe, eastern Asia (mainly China), eastern North America (mainly south-eastern Canada and eastern America), southern Africa, and southern South America. With climate change, the northern boundary of very favourable regions would expand by approximately 2° in Asia, southern Europe, and North America.
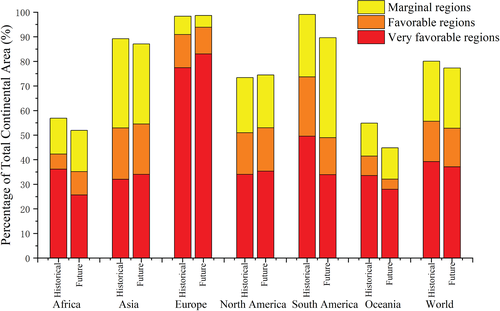
Exactly 16.43% and 15.68% of the total land area (except Antarctica) were considered favourable under historical and future climate conditions. Favourable land was generally distributed along the margins of very favourable regions. It is distributed as a band across the Eurasian continent and Canada, and is also scattered in western North America, central and southern South America, central Asia, and southern Africa. The range of favourable regions also shows an obvious northward shift compared with that of historical climate conditions, with both the south boundary and north boundary moving by approximately 2° in northern Asia, Europe, and North America. In southern South America, favourable regions became marginal. In central Africa, very favourable regions became favourable.
Marginal regions represent 24.44% and 24.54% of the total land area under historical and future climate conditions, respectively. This area was mainly distributed from 60°N to 70°N in northern Asia, North America, and Western China. The highest marginal percentage was 36.32% of Asia's continental land area under historical climate conditions, and 40.65% of South America's continental land area under future climate conditions. Large very favourable and favourable regions in southern South America would become marginal regions, leading to an increase of 15.25% in marginal areas. Slight changes in marginal regions also occurred in southern and central Africa, and northern and central Oceania.
On the whole, climate change would lead to a net 2.77% decrease in the area of climate-suitable regions for ALB.
The effect of climate change on ALB's climate suitability
The suitability of habitable regions for ALB, indicated by EI values, would change to varying extents with climate change (Fig. 4A). Increased climate suitability would mainly occur north of 50°N in the Northern Hemisphere, and would include the entirety of Canada and Russia, as well as most of Europe. Most of Western China may also become more suitable for ALB survival. By contrast, the climate-suitable regions in southern North America, South America, Africa, southern Asia, and inner Oceania would become less suitable for ALB. Dramatic EI value declines in south-eastern North America, northern South America, and southern Africa indicate that ALB is more sensitive to climate change in these areas. Regions in northern Africa, coastal Southeast Asian countries, and most of Oceania are unfavourable for ALB under both historical and future climate conditions.
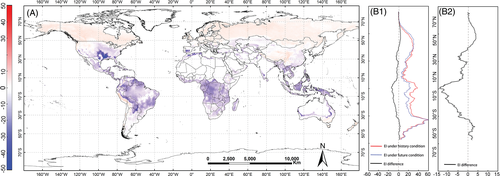
EI values under both historical and future climate conditions are greater at 40°N, the equator, and 40°S, with their peak values distributed approximately symmetrically around the equator (Fig. 4B-1). The most pronounced difference in EI value from historical to future climate scenarios occurred near the equator. At low latitudes (around 45°N and 30°S), EI values generally decrease from present to future climate scenarios, and generally increase at middle to high latitudes (north of 45°N and south of 30°S). There was a greater decrease in EI values than increase (Fig. 4B-2).
Effects of hosts on ALB potential distribution
Known distribution of hosts
Most optimum hosts were distributed north of 10°N in North America, Europe, and Asia, and the rest were located sporadically in the coastal areas of South America, the southernmost zones of Africa, and the southeast zones of Oceania (Fig. 5B). Optimum hosts were much more abundant in the Northern Hemisphere than in the Southern Hemisphere. Sub-optimum hosts were also widely distributed in the Northern Hemisphere, and had a vast distribution in coastal areas in the Southern Hemisphere (Fig. 5C). Occasional to rare hosts in the Southern Hemisphere were located throughout Oceania, most of South America, and nearly half of Africa, where they extended further inland compared with sub-optimum and optimum hosts (Fig. 5D). Occasional to rare hosts had an equally vast distribution in the Northern Hemisphere.
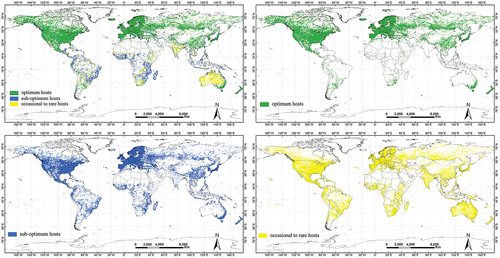
Optimum hosts play a dominant role in the Northern Hemisphere compared with sub-optimum and occasional to rare hosts; the latter two are much more abundant in the Southern Hemisphere. Overall, there was much overlap in distribution of the three host types, and they cumulatively cover the majority of Earth's surface (apart from Antarctica).
Effects of hosts on ALB potential distribution
There was little difference between the potential distribution maps under historical and future climate conditions, with minor changes in northern South America and central Africa (Fig. 6). In both climate scenarios, hosts were present in most areas that were climate-suitable for ALB, and there is more overlap between climatically favourable regions and host distribution in the Northern Hemisphere than in the Southern Hemisphere.
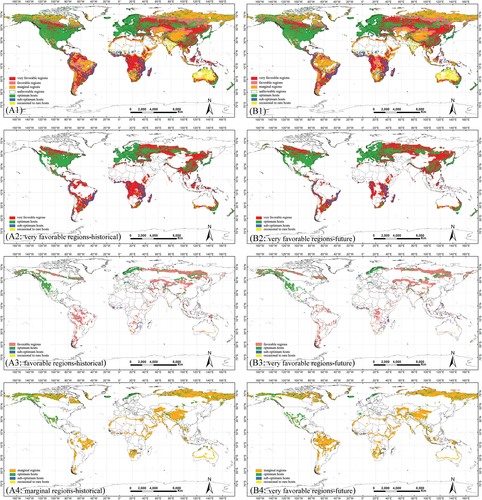
In general, host cover of very favourable regions in the Northern Hemisphere was much greater than that of the Southern Hemisphere. Regions throughout North America, Europe, and Asia have excellent conditions for ALB survival both in terms of climatic and host suitability. By contrast, a few of the very favourable regions without covering any hosts mainly located in South America and Africa, and the others also scattered around 50°N in the Northern Hemisphere, such as eastern Europe, north-east China (Fig. 6A-2, B-2).
Most climatically favourable regions in North America, Europe, and parts of Asia overlapped with the distribution of optimum hosts. Climatically favourable regions to the south of 10°N were mainly filled with sub-optimum and occasional to rare hosts. Some favourable regions in western Russia, Kazakhstan, Mongolia, America, central South America, and the northern boundary of Africa were also without hosts (Fig. 6A-3, B-3).
Climatically marginal regions overlapped with optimum hosts in North America, Europe, and parts of Asia. Similar to the favourable regions in the south of 10°N, these marginal regions also overlapped sporadically with sub-optimum and occasional to rare hosts. Areas with marginal climatic suitability and no hosts were found in the northern boundary and central region of Africa, eastern South America, north-eastern Russia, Western China, the coastal areas of Saudi Arabia, and the confluence of Kazakhstan, Uzbekistan, Kyrgyzstan, and Afghanistan (Fig. 6A-4, B-4).
Discussion
With climate as the only consideration, our results indicated that a large area of land in all continents (except Antarctica) was vulnerable to ALB invasion under both historical and future climate conditions (Fig. 2). The Southern Hemisphere would be more suitable for ALB survival because its average EI values were greater than those of their latitudinal counterparts in the Northern Hemisphere (Fig. 4B-1), even though climate change would lead to significant decrease of climate suitability in southern latitude. By contrast, future ALB invasion potential in the Southern Hemisphere would greatly decrease if hosts are taken into consideration (Fig. 4). Most regions in South America, Africa, and Oceania would not be suitable for ALB survival owing to the lack of hosts. For example, Acer genus, which are one of its preferred hosts, mainly located in the northern temperate regions, and the sporadic distribution in the Southern Hemisphere is only limited in the north edge of Africa (Tingzhi, 1998). Optimum hosts would instead be widely distributed in the Northern Hemisphere (Fig. 5). The possibility of ALB outbreak in the Northern Hemisphere is much higher than that in the Southern Hemisphere under future climates due to the abundant host cover, just as MacLeod et al. (2002) thought the establishment of ALB in Europe would not be inhibited because of the suitable climate and contiguous host trees throughout the continent, which also benefits the proximity of the sites of that hosts and of adults. Instead, the scattered distribution of hosts in the Southern Hemisphere does not appear conducive to the dispersal of ALB owing to the disruption of natural spread paths. These results further confirmed the necessity of taking the hosts into account when predicting the potential distribution of pests.
Several methods have been adopted to consider the effects of hosts in SDMs: Berzitis et al. (2014) and Zou et al. (2020) used host plant availability; Shatz et al. (2015) applied a host probability variable to the predictive model. However, neither of those are applicable for the polyphagous ALB because their host preference and suitability vary among populations in different regions (Meng et al., 2015; Faccoli et al., 2015b). ALB's host range also expands when the pest migrates to new areas (Morewood et al., 2004). For our study, the application of host occurrence records to both historical and future climate conditions was the most effective method of emulating host effect on ALB distribution. One aspect that was not included in our analysis was how climate change could affect the distribution and abundance of hosts. The hosts are influenced by many nonclimatic factors, such as human activities, soil properties, etc. Thus, the future distributions of host plants are more difficult to predict accurately with SDMs than climate change effects on animals. Another deficiency of the present method was that the landscape patterns and intrinsic properties of hosts were neglected. The two factors may also alter the distribution of ALB. The hosts planted in different habitats differ in suitability and functional connectivity for ALB, and their growth status also determines the attraction to the pest (Ding et al., 2011; Faccoli et al., 2015a).
The potential distribution of ALB has been studied extensively using various SDMs; owing to differences in model selection, parameter settings, and climate data, our findings differ from those of some previous studies (MacLeod et al., 2002; Peterson & Scachetti-Pereira, 2004; Shatz et al., 2015). These studies have focused on specific locations and the risk of invasion under current climate conditions, thus the local area of our global prediction for potential distribution was slightly different compared with their results. For example, our prediction of ALB distribution under historical climates (Fig. 2A) was similar to the results of MacLeod et al. (2002). MacLeod et al. (2002) used CLIMEX to predict the suitable regions for ALB in Europe, and yielded generally similar results with our historical prediction (Fig. 2A) that suitable regions for ALB were distributed throughout southern Europe, but differed from our prediction with respect to precise EI values and ALB distribution in marginal regions. Although the EI values estimated in our study were relatively low, the marginal regions extended northward to Norway, Sweden, and Finland. The differences between studies may be explained by differences in parameters (Table S3). MacLeod et al. (2002) set TTCS to 0°C, compared with −17°C in our study. In addition, the climate data in MacLeod et al.'s study were extracted from European meteorological stations within CLIMEX before 2002, whereas we obtained historical data (1987–2016) from an external data source (CRU) with higher resolution. This difference in the period of climate data analysed may also explain the difference in results. Besides, our projections for the future enable us to investigate the effects of climate change on ALB's distribution, which is absent in all other similar research.
Although SDMs are widely used in estimating potential distributions of species, there remain limitations that can affect their results. One such limitation is their inability to capture variation on a finer scale. In terms of ALB, its voltinism differs among various local climatic conditions and correlates strongly with latitude. The time required for ALB to complete one generation may vary among populations even within the same area, depending on the host in which the larvae develop (Hu et al., 2009). If climate change makes ALB univoltine in a much larger share of host-dominated forest systems, then that could greatly increase its global impact. In addition, biological characteristics such as cold hardiness varied widely between populations (Feng et al., 2014). As an endophytic pest, ALB spends most of its life cycle in a microhabitat buffered against climatic extremes, and variation in this microhabitat is difficult to consistently model (Hu et al., 2009). CLIMEX models are built based on a single series of parameters representing generalized biological characteristics, and the accuracy of parameter estimation relies on the availability of biological information for a species. Thus, other considerations that are ignored when modelling global distribution include the adaptation of geographical populations to local climate, and evolutionary changes caused by climate change (Trewin, 2018).
There are some species-specific limitations in addition to model limitations. ALB is frequently transported in solid wood packing materials that accompany traded goods, which poses a risk to unaffected countries and regions (MacLeod et al., 2002). Shatz et al. (2015) found that though the risk of ALB spread and colonization is highest in suitable regions immediately surrounding the epicentre of an ALB outbreak, areas of lower risk are accessible by long-range dispersal via human transportation networks. The probability of importing new pests via commercial pathways has increased as the volume, speed, and frequency of international trade have increased (Mumford, 2002); it is thus important to include the effects of trade in future models.
Our prediction of the potential distribution of ALB provides theoretical support for the establishment of early monitoring and for strengthening pest quarantine policies. Our results indicated a high risk of ALB invasion to many regions where it has not yet been recorded, including western Russia eastern Canada. Our results also showed that climate change would increase climate suitability in most regions in the Northern Hemisphere and shift suitable regions northward, indicating further ALB-caused ecological and economic losses if effective prevention and eradication programmes are not implemented. Stricter monitoring and defence measures in these regions are needed to prevent invasion and spread.
In future research, we will use ensemble outputs from multiple micromodels to model finer-scale differences between various geographical environments. We will develop more complete models that consider all possible climate factors and factors affecting ALB population spread for more accurate predictions. These analyses can generate valuable information for regions with the potential to benefit from ALB quarantine and prevention measures.
Acknowledgements
This study was supported by ‘The Fundamental Research Funds for the Central Universities’ (No. 2016ZCQ07). We would like to thank Jenny Liu for the helpful suggestions and editing on this manuscript.
Open Research
Data availability statement
Data sharing is not applicable to this article as no new data were created or analyzed in this study.